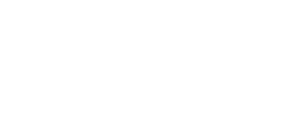
A single-cell atlas of the normal and malformed human brain vasculature
Ethan A. Winkler, Chang N. Kim, Jayden M. Rossjose...
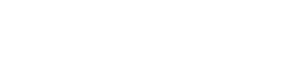
An atlas of cortical arealization identifies dynamic molecular signatures
A. Bhaduri, C. Sandoval-Espinosa, M. Otero-Garcia,...